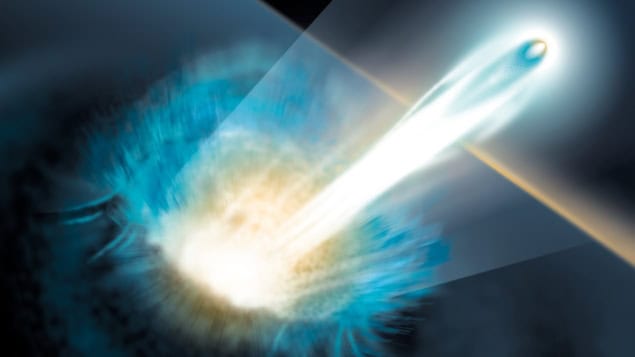
Physicists in Germany say they have passed an important milestone in the development of laser-driven, plasma-based particle acceleration. Proton pulses with energies as high as 150 MeV were created by Tim Ziegler and colleagues at Helmholtz Centre Dresden–Rossendorf (HZDR). This is about 50% higher than the previous record for the technique, and was achieved by better exploiting the temporal profile of laser pulses.
Conventional particle accelerators use radio-frequency cavities to create the high voltages needed to drive particles to near the speed of light. These facilities tend to big; energy hungry; and often require expensive cryogenic cooling. This limits the number of facilities that can be built and where they can be located. If accelerators could be made smaller and less expensive, it would be a boon for applications as diverse as cancer therapies and materials science.
As a result, there is a growing interest in laser-driven plasma-based accelerators, which have the potential to be far more compact and energy efficient that conventional systems.
Ripping away electrons
These accelerators work by firing intense laser pulses into wafer-thin solid targets. The pulse rips away electrons from the target, leaving behind the positively charged atomic cores. This creates a very large voltage difference over a very small distance – which can be used to accelerate pulses of charged particles such as protons.
While these voltage gradients can be much larger than those in conventional accelerators, significant challenges must be overcome before this technique can be used in practical facilities.
“The adoption of plasma-based proton acceleration has been hampered by the slow progress in increasing ion energy,” Ziegler explains. One challenge is that today’s experiments are done at one of just a few high-power, ultrashort-pulse lasers around the world – including HZDR’s DRACO-PW facility. “Firing only a few shots per day, access and availability at these few facilities is constrained,” adds Ziegler.
One curious aspect of the ultrashort laser pulses from DRACO-PW is that some of the light precedes the main pulse. This means that the full power of the laser is not used to ionize the target. But now, Ziegler’s team has turned this shortcoming into an advantage.
Early arrival
“This preceding laser light modifies our particle source – a thin plastic foil – making it transparent to the main laser pulse,” Ziegler explains. “This allows the light of the main pulse to penetrate deeper into the foil and initiates a complex cascade of plasma acceleration mechanisms at ultra-relativistic intensities.”
The researchers tested this approach at DRACO-PW. When they previously to irradiated a solid foil target, the plasma accelerated protons to energies as high as 80 MeV.
In their latest experiment, they irradiated the target with a pulse energy of 22 J, and used the leading portion of the pulse to control the target’s transparency. This time, they accelerated a beam of protons to 150 MeV – almost doubling their previous record.
This accelerated proton beam had two distinct parts: a broadband component at proton energies lower than 70 MeV; and a high-energy component comprising protons travelling in a narrow and well-defined beam.
Linear scaling
“Notably, this high-energy component showed a linear scaling of maximum proton energy with increased laser energy, which is fundamentally different to the square-root scaling of the lower energy component,” Ziegler explains. The experiment also revealed that the degree of transparency in the solid target was strongly connected with its interaction with the laser – providing the team with tight control over the accelerator’s performance.
Laser-accelerated proton irradiation: simulating physical and chemical effects
Ziegler believes the result could pave the way for smarter accelerator systems. “This observed sensitivity to subtle changes in the initial laser-plasma conditions makes this parameter ideal for future studies, which will aim for automated optimization of interaction parameters,” he says.
Now that they have boosted the efficiency of ion acceleration, the researchers are hopeful that laser-driven facilities could be built a fraction of the space and energy requirements of conventional facilities.
This would be particularly transformative in medicine, says Ziegler. “Our breakthrough opens up new possibilities to investigate new radiobiological concepts for precise, gentle tumour treatments, as well as scientific studies in efficient neutron generation and advanced materials analysis.”
The research is described in Nature Physics.
- SEO Powered Content & PR Distribution. Get Amplified Today.
- PlatoData.Network Vertical Generative Ai. Empower Yourself. Access Here.
- PlatoAiStream. Web3 Intelligence. Knowledge Amplified. Access Here.
- PlatoESG. Carbon, CleanTech, Energy, Environment, Solar, Waste Management. Access Here.
- PlatoHealth. Biotech and Clinical Trials Intelligence. Access Here.
- Source: https://physicsworld.com/a/laser-driven-accelerator-benefits-from-clever-use-of-light-pulses/
- :has
- :is
- :not
- :where
- $UP
- 150
- 160
- 22
- 80
- a
- About
- accelerate
- accelerated
- acceleration
- accelerator
- accelerators
- achieved
- Adds
- Adoption
- advanced
- Advanced materials
- ADvantage
- aim
- allows
- almost
- also
- an
- analysis
- and
- applications
- approach
- ARE
- around
- AS
- aspect
- At
- atomic
- Automated
- availability
- away
- BE
- Beam
- been
- before
- behind
- believes
- benefits
- Better
- Big
- boost
- Boosted
- breakthrough
- broadband
- built
- but
- by
- CAN
- Cancer
- card
- cascade
- centre
- challenge
- challenges
- Changes
- charged
- chemical
- colleagues
- compact
- complex
- component
- comprising
- concepts
- conditions
- connected
- control
- conventional
- could
- create
- created
- creates
- curious
- day
- deeper
- Degree
- described
- Development
- difference
- different
- distance
- distinct
- diverse
- done
- doubling
- drive
- efficiency
- efficient
- electrons
- energy
- expensive
- experiment
- experiments
- Explains
- exploiting
- facilities
- Facility
- far
- few
- firing
- foil
- For
- fraction
- from
- full
- fundamentally
- future
- generation
- gentle
- Germany
- gradients
- Growing
- growing interest
- had
- Have
- he
- High
- higher
- hopeful
- HTTPS
- Hungry
- ideal
- if
- important
- in
- Including
- increased
- increasing
- information
- initial
- Initiates
- intense
- interaction
- interest
- into
- investigate
- issue
- IT
- ITS
- jpg
- just
- large
- larger
- laser
- lasers
- latest
- leading
- leaving
- less
- light
- limits
- linear
- located
- lower
- made
- Main
- MAKES
- Making
- materials
- max-width
- maximum
- means
- mechanisms
- medicine
- MEV
- milestone
- more
- much
- must
- narrow
- Nature
- Near
- needed
- New
- now
- number
- of
- often
- ONE
- only
- opens
- optimization
- our
- over
- Overcome
- parameter
- parameters
- particularly
- parts
- passed
- pave
- per
- performance
- physical
- Physics
- Physics World
- Plasma
- plastic
- plato
- Plato Data Intelligence
- PlatoData
- portion
- positively
- possibilities
- potential
- power
- Practical
- preceding
- precise
- previous
- previously
- Profile
- Progress
- property
- protons
- providing
- pulse
- record
- require
- Requirements
- research
- researchers
- result
- Revealed
- Rips
- say
- says
- scaling
- Science
- scientific
- Sensitivity
- shots
- showed
- significant
- simulation
- slow
- small
- smaller
- smarter
- solid
- some
- Source
- Space
- speed
- strongly
- studies
- such
- Systems
- Target
- targets
- team
- technique
- tend
- tested
- than
- that
- The
- the world
- their
- therapies
- There.
- These
- they
- thin
- this
- those
- thumbnail
- time
- to
- today’s
- track
- transformative
- Transparency
- transparent
- treatments
- true
- Turned
- two
- use
- used
- very
- Voltage
- was
- Way..
- WELL
- well-defined
- were
- when
- which
- will
- with
- Work
- world
- would
- zephyrnet