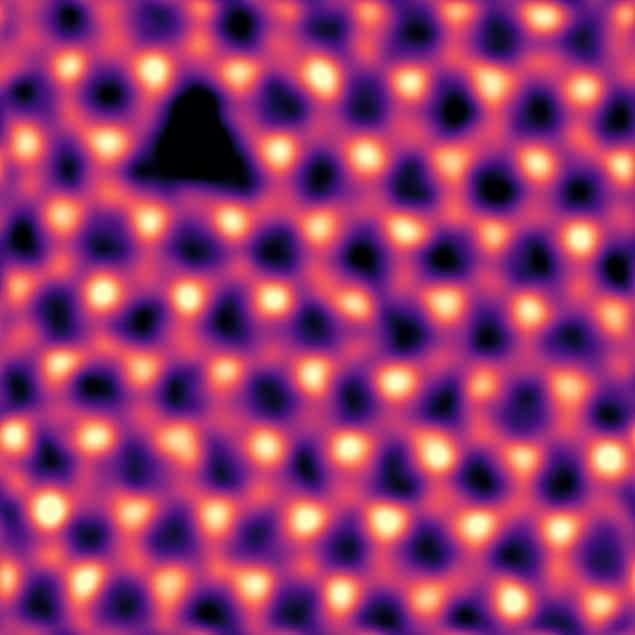
A beam of electrons can “kick” single atoms out of a two-dimensional sheet of hexagonal boron nitride (hBN) in a controllable way, defying predictions that electron irradiation would be too damaging for this purpose. Even more remarkably, the physicists behind the discovery predict that a higher-energy version of the same technique could preferentially remove nitrogen atoms from the hBN lattice, which is unexpected since nitrogen is heavier than boron. The empty spaces, or vacancies, left behind by the “missing” nitrogen atoms could have applications in quantum computing, communication networks and sensors.
Nitrogen vacancies in hBN have optical properties that make them ideal for use in emerging quantum and optoelectronic devices. The downside is that they can be difficult to isolate, but researchers at the University of Vienna led by experimental physicist Toma Susi have now found a way to do it using a technique called aberration-corrected scanning transmission electron microscopy (TEM).
“Transmission electron microscopy allows us to image the atomic structure of materials and it is particularly well suited to directly reveal any defects in the lattice of the sample,” explains Susi. “Aberration correction provides us with the resolution to observe single atoms – it’s like using eyeglasses to see more clearly – but it can also be used to remove these atoms.”
Previously, TEM measurements were usually conducted under relatively poor vacuum conditions. In these circumstances, the gas molecules that remained in the instrument could easily damage hBN samples by etching away atoms in the material’s crystalline lattice. The high-energy electron beam can also damage the sample via elastic collisions with the electrons in the beam or electronic excitations.
Lattice damage is greatly reduced
Susi and colleagues overcame these problems by operating the TEM in near ultrahigh vacuum conditions and testing different electron-beam energies between 50 and 90 keV. They found that the lack of residual gas molecules under the improved vacuum suppresses unwanted etching effects, which occur extremely fast and would otherwise prevent single atoms from being controllably removed.
What is more, the team found that the TEM could create single vacancies of either boron and nitrogen at intermediate energies. Although boron is twice as likely to be ejected at energies below 80 keV because of its lower mass, at higher energies, the team predict that nitrogen will become easier to eject, thus allowing this vacancy to be preferentially created. “To create these vacancies, nothing special is needed,” Susi tells Physics World. “The electrons used for imaging have enough energy to knock out atoms in the hBN lattice.”
The fact that the researchers performed measurements over many electron energies allowed them to collect robust statistics on how the missing atoms are generated, something that will be useful for developing a future theory for how vacancies can be created using a TEM.
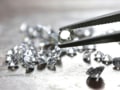
The diamond quantum revolution
“Now that we are able to predict how much we need to irradiate the material at each energy to kick out nitrogen or boron atoms, we can design experiments that optimize the desired distribution of vacancies,” Susi says. “We’ve also pioneered atomic-level manipulation by directing the electron beam at individual lattice sites.
“We previously thought hexagonal boron nitride would damage too quickly to be suitable for such treatment. We’ll have to reconsider that now.”
Susi says that the next step will be to generalize the results beyond hBN. “With better theoretical models, we could predict how the beam interacts not only with hBN but potentially other materials, such as graphene and bulk silicon,” he says.
The researchers detail their work in Small.
- SEO Powered Content & PR Distribution. Get Amplified Today.
- PlatoData.Network Vertical Generative Ai. Empower Yourself. Access Here.
- PlatoAiStream. Web3 Intelligence. Knowledge Amplified. Access Here.
- PlatoESG. Automotive / EVs, Carbon, CleanTech, Energy, Environment, Solar, Waste Management. Access Here.
- BlockOffsets. Modernizing Environmental Offset Ownership. Access Here.
- Source: https://physicsworld.com/a/electron-kick-removes-single-atoms-from-2d-material/
- :is
- :not
- 2D
- 50
- 80
- a
- Able
- allowed
- Allowing
- allows
- also
- Although
- and
- any
- appears
- applications
- ARE
- AS
- At
- away
- BE
- Beam
- because
- become
- behind
- being
- below
- Better
- between
- Beyond
- but
- by
- called
- CAN
- circumstances
- clearly
- colleagues
- collect
- Communication
- computing
- conditions
- conducted
- could
- create
- created
- damaging
- Dark
- Design
- desired
- detail
- developing
- Devices
- Diamond
- different
- difficult
- directing
- directly
- discovery
- distribution
- do
- downside
- each
- easier
- easily
- effects
- either
- Electronic
- electrons
- emerging
- energy
- enough
- Even
- experiments
- Explains
- extremely
- fact
- FAST
- For
- found
- from
- future
- GAS
- generated
- Graphene
- greatly
- Have
- he
- higher
- How
- http
- HTTPS
- ideal
- image
- Imaging
- improved
- in
- individual
- information
- instrument
- interacts
- issue
- IT
- ITS
- jpg
- kick
- Lack
- Led
- left
- like
- likely
- lower
- make
- Manipulation
- many
- Mass
- material
- materials
- max-width
- measurements
- Microscopy
- missing
- models
- more
- much
- Near
- Need
- needed
- networks
- next
- nothing
- now
- observe
- of
- on
- only
- operating
- Optimize
- or
- Other
- otherwise
- out
- over
- particularly
- performed
- Physics
- Physics World
- pioneered
- plato
- Plato Data Intelligence
- PlatoData
- poor
- potentially
- predict
- Predictions
- prevent
- previously
- problems
- properties
- provides
- purpose
- Quantum
- quantum computing
- quickly
- reconsider
- relatively
- remained
- remove
- Removed
- researchers
- Resolution
- Results
- reveal
- robust
- same
- says
- scanning
- see
- sensors
- sheet
- Silicon
- since
- single
- Sites
- something
- spaces
- special
- Spot
- statistics
- Step
- structure
- such
- suitable
- team
- tells
- Testing
- than
- that
- The
- their
- Them
- theoretical
- theory
- These
- they
- this
- thought
- thumbnail
- to
- too
- top
- treatment
- true
- Twice
- under
- Unexpected
- university
- unwanted
- us
- use
- used
- using
- usually
- Vacuum
- version
- via
- visible
- Way..
- we
- WELL
- were
- which
- will
- with
- Work
- world
- would
- zephyrnet