Physicist Laura Marini is run co-ordinator and site manager of the Cryogenic Underground Observatory for Rare Events (CUORE). Operated by an international collaboration, the experiment is situated deep beneath a mountain in Italy’s Abruzzo region at the Gran Sasso National Laboratory of the National Institute of Nuclear Physics. Marini received a PhD in physics from the University of Genoa in 2018 and then did a postdoc at the University of California, Berkeley. She began working on CUORE during her PhD and today she is affiliated with Italy’s Gran Sasso Science Institute and the Gran Sasso lab. Marini spoke to Richard Blaustein about her role at CUORE and the experiment’s recent milestone in its ongoing investigation of whether neutrinos are Majorana particles.
Can you describe your dual role at CUORE?
Right now, I am run co-ordinator for this current experiment and site manager for CUORE. As run co-ordinator, I make sure that the experiment keeps running without stopping. This is important because we are looking for extremely rare events, so we want to take data for as long as possible without stopping. I work on both the cryogenic part of the experiment and the data collection part. I also work on minimizing the background noise level in the experiment – which is also important when looking for rare events.
My site manager role is a bit broader than run co-ordinator. I handle the interface between the experiment and Gran Sasso National Laboratory, coordinate onsite activities and organize the maintenance of all the systems and subsystems.
Can you describe CUORE and what it is seeking to measure?
CUORE looks for rare events in physics and it was specifically designed to search for neutrinoless double beta decay. This process is expected to occur if neutrinos are their own anti-particles – that is, if they are Majorana particles. Answering this question is important because if neutrinos are proven to be Majorana particles, the mystery of why neutrino masses are so small within the Standard Model of particle physics will be solved.
We search for neutrinoless double beta decay in the isotope tellurium-130 because it is known to undergo ordinary double beta decay and it has a high natural abundance. CUORE has 184 tellurium dioxide crystals that are kept near 10 mK inside a large cryostat. The cryostat does not use liquid helium but rather has five pulse tube cryocoolers.
The experiment must be kept at a very low temperature because we search for neutrinoless double beta decay by detecting the tiny increase in temperature within a crystal that occurs because of the decay. Before CUORE, only a small experimental volume and mass could be cooled but we have increased this tremendously by cooling up to 1.5 tonne of material at base temperature. Another advantage of CUORE is that the experiment has very good energy resolution and operates over a very broad energy range – which should help it identify decay events.
What is the significance of CUORE’s recent achievement of acquiring a “tonne–year” of data?
Tonne-year refers to the mass of the tellurium oxide being monitored multiplied by the length of time that the experiment collected data. The mass is 741 kg and data were acquired in runs that were done between 2017 and 2020. Not every run involved using the entire mass, but all together one tonne–year worth of data were collected
There are two significant aspects to this. First, this is the first time that such a large mass has been cooled in a cryostat. Second, because we were able run the experiment for such a long time, we have shown that cryogenic calorimeters are a viable way to search for neutrinoless double beta decay.
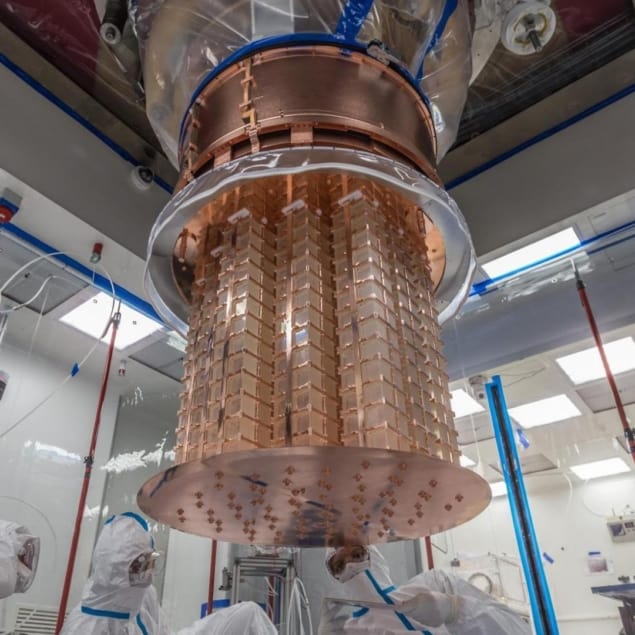
What did this tonne-year of data tell you and your colleagues?
To be clear, we have not found Majorana particles. Instead, we have been able to set a lower limit on the half-life of neutrinoless double beta decay. We now know that the half-life is greater than 2.2×1025 years. We can conclude this because if the half-life was shorter, we would have expected to see at least one or more events in CUORE.
Can CUORE be used to explore other areas of physics?
Yes. CUORE is designed to search for rare events and therefore it has the potential to look for dark matter. Dark matter particles are expected to interact with CUORE’s detector materials very rarely and this would involve the release of very small amounts of energy. So, the search for dark matter would benefit from the experiment’s large mass and long run time. A dark matter search would involve exploring another energy region in the detector and there are groups of physicists within the CUORE collaboration looking at that possibility.
Does CUORE’s cryogenic milestone have some bearing on quantum computing?
I am not an expert in quantum computing, but generally, solid state devices that process quantum information require long quantum coherence times. We know that heat and cosmogenic radiation both reduce quantum coherence times. Running experiments underground with advanced cryogenics offers protection from these negative effects. While CUORE’s tellurium dioxide crystals cannot be used for quantum computing, the fact that we have achieved such a long experimental run underground with a very large cryostat and with clean materials could be potentially very useful for the development of quantum technologies.
What will the future bring for the CUORE collaboration?
CUORE will run until 2024 and we are already working on the CUORE Upgrade with Particle Identification – or CUPID. We will replace CUORE’s current tellurium dioxide crystals with lithium molybdate crystals. When particles produced in neutrinoless double beta decay interact with lithium molybdate, they produce both heat and light. This light will be detected along with the heat, and the ratio of heat-to-light will allow us to reject background events involving particles that are not produced by neutrinoless double beta decay. The cryogenic structure of the experiment will also be upgraded.