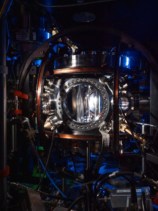
Physicists at the University of Colorado, Boulder, US have determined the shape of the electron’s charge distribution to unprecedented precision. Led by Eric Cornell and Jun Ye, the team found that any imbalance in this charge distribution – the electron’s electric dipole moment, or eEDM – must be less than 4.1 x 10-30 e cm, with an uncertainty of 2.1×10-30 e cm. This precision is equivalent to measuring the size of the Earth to within the dimensions of a virus, and the result has important implications in the search for new particles beyond the Standard Model.
One way to look for new particles is to do it directly, by smashing known particles together in large particle accelerators such as the Large Hadron Collider (LHC) at ever increasing energies. The alternative is to do it indirectly, by looking for tell-tale signs of new particles in the charge distribution of the electron. This is the method the CU-Boulder team employed, and it allows the search to be carried out on a laboratory tabletop.
The symmetry of the universe, mirrored in an electron
The electron has a magnetic moment due to its spin, and can be thought of as a rotating charge generating a magnetic dipole. In contrast, an electric dipole moment (EDM) could only occur if the charge distribution of the electron is distorted slightly. The presence of such a distortion would mean that the electron no longer obeys time-reversal symmetry, which is the fundamental requirement that physics is the same whether time flows forwards or backwards.
To understand why this symmetry would be violated, consider what would happen if time reversed. The electron would then spin the opposite way and the direction of its magnetic moment would flip. The eEDM, however, is a result of a permanent charge distortion, so it would remain unchanged. This is a problem, because if we start with both moments parallel, a time reversal leads to them being antiparallel, violating time symmetry.
The Standard Model – the current best framework for the forces and particles that make up the universe – only allows for a very small amount of time-symmetry violation, so it predicts that the electron’s electric dipole moment cannot be more than ~10-36 e cm. This is much too small to be experimentally testable even with current state-of-the-art equipment.
However, extensions to the Standard Model such as supersymmetry predict the existence of many new particles at energies higher than any discovered so far. These new particles would interact with the electron to give it a much larger eEDM. Searching for a non-zero eEDM is therefore a search for new physics beyond the Standard Model and a hunt for a “marker” of new particles.
Molecular ions help measure the eEDM
To measure the eEDM, the CU-Boulder researchers detect how an electron wobbles in an external magnetic and electric field. This wobble, or precession, is similar to the rotation of a gyroscope in a gravitational field. When an electron is placed inside a magnetic field, it will precess at a specific frequency thanks to its magnetic moment. If the electron also has an EDM, applying an electric field will change this rate of precession: if the electron is orientated in one direction with respect to the electric field, the frequency of precession will speed up; if it’s “pointing” in the other direction, the rate will slow down.
“We are able to determine the eEDM by measuring the frequency difference of this wobble, once with the electron oriented in one direction and again with it in the other,” explains Trevor Wright, a PhD student at CU-Boulder and co-author of a paper in Science outlining the results.
Rather than study an electron on its own, the researchers monitor the precession frequency of an electron inside hafnium fluoride molecular ions (HfF+). The internal electric field of these ions makes the frequency difference much larger, and by confining the ions in a trap, the researchers were able to measure the precession of the electron for up to three seconds, Trevor explains. Indeed, the researchers had such good control over the molecules that they were able to measure the precession frequency to a precision of tens of µHz.
After 620 hours of data collection, during which the researchers changed multiple experimental parameters to investigate and reduce systematic errors, they reduced the upper limit on the electron EDM to 4.1×10-30 e cm. This is 37 times smaller than their own previous measurement and 2.4 times smaller than the previous best limit.
David vs. Goliath; eEDM vs LHC
The new limit contradicts predictions for the eEDM made by some extensions to the Standard Model such as split supersymmetry (split SUSY) and spin-10 grand unified theory, though the previous limit had already given them a thumbs-down. As team member Luke Caldwell, a postdoctoral researcher at CU-Boulder, explains: “Typically the predicted size of the eEDM scales inversely with the energy scale of the proposed new physics and so more precise measurements of the eEDM probe physics at higher and higher energy scales. Our measurement provides constraints on new physics at energy scales at tens of TeV, well beyond the reach of particle colliders like the LHC.” This makes it unlikely that new particles exist below these energies.
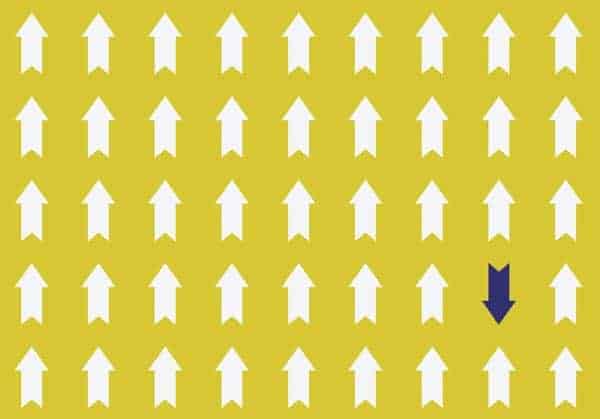
Measuring (almost) zero
Many researchers, including the team at Boulder, are pushing to lower the limit even further. “The next generation of the eEDM experiment will use a different molecule, thorium fluoride. This molecule is inherently more sensitive to the eEDM,” says Caldwell, adding that they should be able to measure its electron precession for 10-20s. “A prototype of this new apparatus is already up and running, trapping ions and recording the first electron precessions.”
- SEO Powered Content & PR Distribution. Get Amplified Today.
- PlatoData.Network Vertical Generative Ai. Empower Yourself. Access Here.
- PlatoAiStream. Web3 Intelligence. Knowledge Amplified. Access Here.
- PlatoESG. Automotive / EVs, Carbon, CleanTech, Energy, Environment, Solar, Waste Management. Access Here.
- PlatoHealth. Biotech and Clinical Trials Intelligence. Access Here.
- ChartPrime. Elevate your Trading Game with ChartPrime. Access Here.
- BlockOffsets. Modernizing Environmental Offset Ownership. Access Here.
- Source: https://physicsworld.com/a/physicists-measure-the-electron-electric-dipole-moment-to-unprecedented-precision/
- :has
- :is
- $UP
- 1
- a
- Able
- accelerators
- adding
- again
- allows
- already
- also
- alternative
- amount
- an
- and
- any
- Applying
- ARE
- AS
- At
- BE
- because
- being
- below
- BEST
- Beyond
- both
- by
- CAN
- cannot
- carried
- casey
- Chamber
- change
- changed
- charge
- click
- Co-Author
- collection
- Colorado
- Consider
- constraints
- contrast
- control
- could
- Current
- data
- detect
- Determine
- determined
- difference
- different
- dimensions
- direction
- directly
- discovered
- distribution
- do
- down
- due
- during
- e
- earth
- Electric
- employed
- energy
- equipment
- Equivalent
- Errors
- Even
- EVER
- exist
- existence
- experiment
- experimental
- Explains
- extensions
- external
- far
- field
- First
- Flip
- Flows
- For
- Forces
- found
- Framework
- Frequency
- fundamental
- further
- generating
- generation
- Give
- given
- good
- gravitational
- had
- happen
- Have
- help
- higher
- HOURS
- How
- However
- HTTPS
- hunt
- if
- image
- imbalance
- implications
- important
- in
- Including
- increasing
- indeed
- indirectly
- information
- inherently
- inside
- interact
- internal
- investigate
- issue
- IT
- ITS
- jpg
- known
- laboratory
- large
- larger
- Leads
- Led
- less
- like
- LIMIT
- longer
- Look
- looking
- lower
- made
- Magnetic field
- make
- MAKES
- many
- max-width
- mean
- measure
- measurement
- measurements
- measuring
- member
- method
- model
- molecular
- molecule
- moment
- Moments
- Monitor
- more
- much
- multiple
- must
- New
- next
- no
- of
- on
- once
- ONE
- only
- open
- opposite
- or
- Other
- our
- out
- over
- own
- Paper
- Parallel
- parameters
- permanent
- phd
- photo
- Physics
- Physics World
- plato
- Plato Data Intelligence
- PlatoData
- precise
- Precision
- predict
- predicted
- Predictions
- Predicts
- presence
- previous
- probe
- Problem
- proposed
- prototype
- provides
- Pushing
- Rate
- reach
- recording
- reduce
- Reduced
- remain
- requirement
- researcher
- researchers
- respect
- result
- Results
- Reversal
- running
- same
- says
- Scale
- scales
- Science
- Search
- searching
- seconds
- sensitive
- Shape
- should
- Signs
- similar
- Size
- slow
- small
- smaller
- So
- so Far
- some
- specific
- speed
- Spin
- split
- standard
- start
- state-of-the-art
- Student
- Study
- such
- team
- tens
- than
- Thanks
- that
- The
- their
- Them
- then
- theory
- therefore
- These
- they
- this
- thorium
- though?
- thought
- three
- thumbnail
- time
- times
- to
- together
- too
- trapping
- Trevor
- true
- Uncertainty
- understand
- unified
- Universe
- university
- unlikely
- unprecedented
- us
- use
- used
- Vacuum
- very
- violated
- Violating
- VIOLATION
- virus
- vs
- Way..
- we
- WELL
- were
- What
- when
- whether
- which
- why
- will
- with
- within
- world
- would
- X
- zephyrnet