1Centre for Engineered Quantum Systems, University of Queensland, QLD 4072, Australia
2School of Mathematics and Physics, University of Queensland, QLD 4072, Australia
3Department of Electrical, Computer and Energy Engineering, University of Colorado Boulder, Boulder, Colorado 80309, USA
4National Institute of Standards and Technology, 325 Broadway, Boulder, Colorado 80305, USA
5Quantum Valley Ideas Lab, Waterloo, ON N2L 6R2, Canada
Find this paper interesting or want to discuss? Scite or leave a comment on SciRate.
Abstract
Superconducting transition-edge sensors (TES) are extremely sensitive microcalorimeters used as photon detectors with unparalleled energy resolution. They have found application from measuring astronomical spectra through to determining the quantum property of photon-number, $hat{n} {=} hat{a}^† hat{a}$, for energies from 0.6-2.33eV. However, achieving optimal energy resolution requires considerable data acquisition – on the order of 1GB/min – followed by post-processing, which does not allow access to energy information in real time. Here we use a custom hardware processor to process TES pulses while new detections are still being registered, allowing photon-number to be measured in real time as well as reducing data requirements by orders-of-magnitude. We resolve photon number up to $n=16$ – achieving up to parts-per-billion discrimination for low photon numbers on the fly – providing transformational capacity for applications of TES detectors from astronomy through to quantum technology.
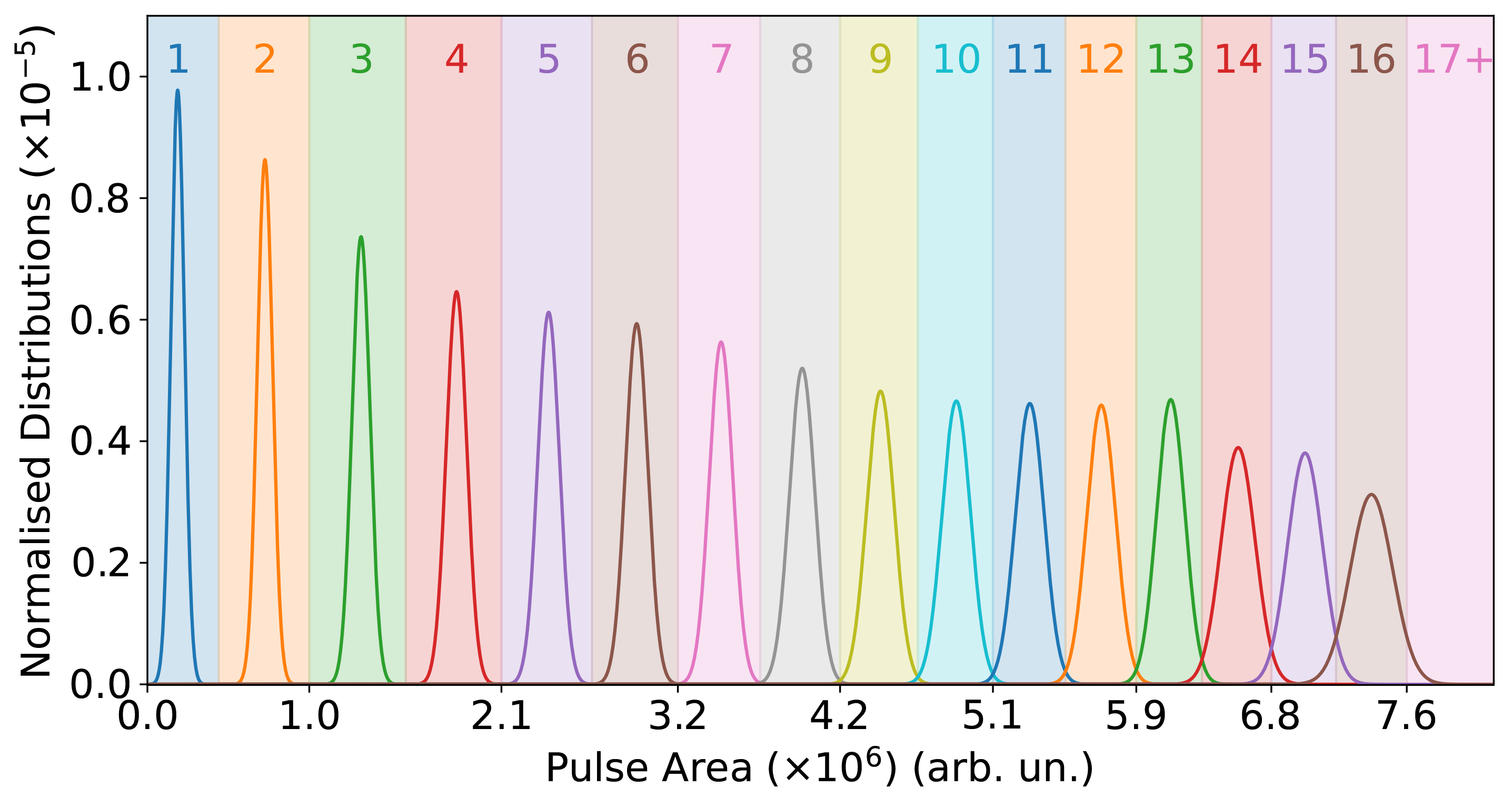
Featured image: Area distribution for assigning photon number $n$ determined by colour-coded thresholds. E.g., centred at $n=2$, the probability to assigned this event to 2 photons is precise to parts-per-billion.
Popular summary
► BibTeX data
► References
[1] R. W. Romani, A. J. Miller, B. Cabrera, E. Figueroa-Feliciano, S. W. Nam, First Astronomical Application of a Cryogenic Transition Edge Sensor Spectrophotometer, ApJ 521 (2) (1999) L153–L156. doi:10.1086/312202.
https://doi.org/10.1086/312202
[2] J. Burney, T. Bay, J. Barral, P. Brink, B. Cabrera, J. Castle, A. Miller, S. Nam, D. Rosenberg, R. Romani, A. Tomada, Transition-edge sensor arrays for UV-optical-IR astrophysics, Nucl. Instrum. Methods Phys. Res. A 559 (2) (2006) 525–527. doi:10.1016/j.nima.2005.12.060.
https://doi.org/10.1016/j.nima.2005.12.060
[3] S. J. Smith, J. S. Adams, S. R. Bandler, J. A. Chervenak, A. M. Datesman, M. E. Eckart, F. M. Finkbeiner, R. Hummatov, R. L. Kelley, C. A. Kilbourne, A. R. Miniussi, F. S. Porter, J. E. Sadleir, K. Sakai, N. A. Wakeham, E. J. Wassell, Multiabsorber transition-edge sensors for x-ray astronomy, JATIS 5 (2) (2019) 021008. doi:10.1117/1.JATIS.5.2.021008.
https://doi.org/10.1117/1.JATIS.5.2.021008
[4] J. L. O’Brien, A. Furusawa, J. Vučković, Photonic quantum technologies, Nat. Photon. 3 (12) (2009) 687–695. doi:10.1038/nphoton.2009.229.
https://doi.org/10.1038/nphoton.2009.229
[5] R. H. Hadfield, Single-photon detectors for optical quantum information applications, Nat. Photon. 3 (12) (2009) 696–705. doi:10.1038/nphoton.2009.230.
https://doi.org/10.1038/nphoton.2009.230
[6] S. Slussarenko, G. J. Pryde, Photonic quantum information processing: A concise review, Appl. Phys. Rev. 6 (1) (2019) 041303. doi:10.1063/1.5115814.
https://doi.org/10.1063/1.5115814
[7] N. Quesada, L. G. Helt, J. Izaac, J. M. Arrazola, R. Shahrokhshahi, C. R. Myers, K. K. Sabapathy, Simulating realistic non-Gaussian state preparation, Phys. Rev. A 100 (2) (2019) 022341. doi:10.1103/PhysRevA.100.022341.
https://doi.org/10.1103/PhysRevA.100.022341
[8] D. Su, C. R. Myers, K. K. Sabapathy, Conversion of Gaussian states to non-Gaussian states using photon-number-resolving detectors, Phys. Rev. A 100 (5) (2019) 052301. doi:10.1103/PhysRevA.100.052301.
https://doi.org/10.1103/PhysRevA.100.052301
[9] I. Tzitrin, J. E. Bourassa, N. C. Menicucci, K. K. Sabapathy, Progress towards practical qubit computation using approximate Gottesman-Kitaev-Preskill codes, Phys. Rev. A 101 (3) (2020) 032315. doi:10.1103/PhysRevA.101.032315.
https://doi.org/10.1103/PhysRevA.101.032315
[10] B. Cabrera, R. M. Clarke, P. Colling, A. J. Miller, S. Nam, R. W. Romani, Detection of single infrared, optical, and ultraviolet photons using superconducting transition edge sensors, Appl. Phys. Lett. 73 (6) (1998) 735–737. doi:10.1063/1.121984.
https://doi.org/10.1063/1.121984
[11] A. J. Miller, S. W. Nam, J. M. Martinis, A. V. Sergienko, Demonstration of a low-noise near-infrared photon counter with multiphoton discrimination, Appl. Phys. Lett. 83 (4) (2003) 791–793. doi:10.1063/1.1596723.
https://doi.org/10.1063/1.1596723
[12] D. Rosenberg, A. Lita, A. Miller, S. Nam, Noise-free high-efficiency photon-number-resolving detectors, Phys. Rev. A 71 (6) (2005) 061803(R). doi:10.1103/PhysRevA.71.061803.
https://doi.org/10.1103/PhysRevA.71.061803
[13] A. E. Lita, A. J. Miller, S. W. Nam, Counting near-infrared single-photons with 95% efficiency, Opt. Express 16 (5) (2008) 3032. doi:10.1364/OE.16.003032.
https://doi.org/10.1364/OE.16.003032
[14] D. Fukuda, G. Fujii, T. Numata, K. Amemiya, A. Yoshizawa, H. Tsuchida, H. Fujino, H. Ishii, T. Itatani, S. Inoue, T. Zama, Titanium-based transition-edge photon number resolving detector with 98% detection efficiency with index-matched small-gap fiber coupling, Opt. Express 19 (2) (2011) 870. doi:10.1364/OE.19.000870.
https://doi.org/10.1364/OE.19.000870
[15] L. Stasi, G. Gras, R. Berrazouane, M. Perrenoud, H. Zbinden, F. Bussières, Fast high-efficiency photon-number-resolving parallel superconducting nanowire single-photon detector, Phys. Rev. Appl. 19 (2023) 064041. doi:10.1103/PhysRevApplied.19.064041. https://doi.org/10.1103/PhysRevApplied.19.064041.
https://doi.org/10.1103/PhysRevApplied.19.064041
[16] C. Cahall, K. L. Nicolich, N. T. Islam, G. P. Lafyatis, A. J. Miller, D. J. Gauthier, J. Kim, Multi-photon detection using a conventional superconducting nanowire single-photon detector, Optica 4 (12) (2017) 1534–1535. doi:10.1364/OPTICA.4.001534. https://opg.optica.org/optica/abstract.cfm?URI=optica-4-12-1534.
https://doi.org/10.1364/OPTICA.4.001534
https://opg.optica.org/optica/abstract.cfm?URI=optica-4-12-1534
[17] X. Tao, S. Chen, Y. Chen, L. Wang, X. Li, X. Tu, X. Jia, Q. Zhao, L. Zhang, L. Kang, P. Wu, A high speed and high efficiency superconducting photon number resolving detector, Supercond. Sci. Technol. 32 (6) (2019) 064002. doi:10.1088/1361-6668/ab0799.
https://doi.org/10.1088/1361-6668/ab0799
[18] F. Mattioli, Z. Zhou, A. Gaggero, R. Gaudio, R. Leoni, A. Fiore, Photon-counting and analog operation of a 24-pixel photon number resolving detector based on superconducting nanowires, Opt. Express 24 (8) (2016) 9067–9076. doi:10.1364/OE.24.009067.
https://doi.org/10.1364/OE.24.009067
[19] R. Hummatov, A. E. Lita, T. Farrahi, N. Otrooshi, S. Fayer, M. J. Collins, M. Durkin, D. Bennett, J. Ullom, R. P. Mirin, S. Woo Nam, Fast transition-edge sensors suitable for photonic quantum computing, Journal of Applied Physics 133 (23) (2023) 234502. doi:10.1063/5.0149478.
https://doi.org/10.1063/5.0149478
[20] C. Portesi, E. Taralli, L. Lolli, M. Rajteri, E. Monticone, Fabrication and characterization of fast tess with small area for single photon counting, IEEE Transactions on Applied Superconductivity 25 (3) (2015) 1–4. doi:10.1109/TASC.2014.2367455.
https://doi.org/10.1109/TASC.2014.2367455
[21] R. Kobayashi, K. Hattori, S. Inoue, D. Fukuda, Development of a fast response titanium-gold bilayer optical tes with an optical fiber self-alignment structure, IEEE Transactions on Applied Superconductivity 29 (5) (2019) 1–5. doi:10.1109/TASC.2019.2909978.
https://doi.org/10.1109/TASC.2019.2909978
[22] M. Eaton, A. Hossameldin, R. J. Birrittella, P. M. Alsing, C. C. Gerry, H. Dong, C. Cuevas, O. Pfister, Resolution of 100 photons and quantum generation of unbiased random numbers, Nat. Photon. 17 (1) (2023) 106–111. doi:10.1038/s41566-022-01105-9. https://www.nature.com/articles/s41566-022-01105-9.
https://doi.org/10.1038/s41566-022-01105-9
https://www.nature.com/articles/s41566-022-01105-9
[23] Z. H. Levine, T. Gerrits, A. L. Migdall, D. V. Samarov, B. Calkins, A. E. Lita, S. W. Nam, Algorithm for finding clusters with a known distribution and its application to photon-number resolution using a superconducting transition-edge sensor, J. Opt. Soc. Am. B 29 (8) (2012) 2066. doi:10.1364/JOSAB.29.002066.
https://doi.org/10.1364/JOSAB.29.002066
[24] P. C. Humphreys, B. J. Metcalf, T. Gerrits, T. Hiemstra, A. E. Lita, J. Nunn, S. W. Nam, A. Datta, W. S. Kolthammer, I. A. Walmsley, Tomography of photon-number resolving continuous-output detectors, New J. Phys. 17 (10) (2015) 103044. doi:10.1088/1367-2630/17/10/103044.
https://doi.org/10.1088/1367-2630/17/10/103044
[25] B. Calkins, A. E. Lita, A. E. Fox, S. Woo Nam, Faster recovery time of a hot-electron transition-edge sensor by use of normal metal heat-sinks, Appl. Phys. Lett. 99 (24) (2011) 241114. doi:10.1063/1.3659686.
https://doi.org/10.1063/1.3659686
[26] L. Lolli, E. Taralli, M. Rajteri, T. Numata, D. Fukuda, Characterization of Optical Fast Transition-Edge Sensors With Optimized Fiber Coupling, IEEE Trans. Appl. Supercond. 23 (3) (2013) 2100904. doi:10.1109/TASC.2013.2238981.
https://doi.org/10.1109/TASC.2013.2238981
[27] K. D. Irwin, An application of electrothermal feedback for high resolution cryogenic particle detection, Appl. Phys. Lett. 66 (15) (1995) 1998–2000. doi:10.1063/1.113674.
https://doi.org/10.1063/1.113674
[28] G. Gillett, A hardware signal processor for Transition Edge Sensors, PhD Thesis, The University of Queensland (2018). doi:10.14264/uql.2019.51.
https://doi.org/10.14264/uql.2019.51
[29] J. S. Lundeen, A. Feito, H. Coldenstrodt-Ronge, K. L. Pregnell, C. Silberhorn, T. C. Ralph, J. Eisert, M. B. Plenio, I. A. Walmsley, Tomography of quantum detectors, Nat. Phys. 5 (1) (2009) 27–30. doi:10.1038/nphys1133.
https://doi.org/10.1038/nphys1133
[30] A. Feito, J. S. Lundeen, H. Coldenstrodt-Ronge, J. Eisert, M. B. Plenio, I. A. Walmsley, Measuring measurement: theory and practice, New J. Phys. 11 (9) (2009) 093038. doi:10.1088/1367-2630/11/9/093038.
https://doi.org/10.1088/1367-2630/11/9/093038
[31] G. Brida, L. Ciavarella, I. P. Degiovanni, M. Genovese, L. Lolli, M. G. Mingolla, F. Piacentini, M. Rajteri, E. Taralli, M. G. A. Paris, Quantum characterization of superconducting photon counters, New J. Phys. 14 (8) (2012) 085001. doi:10.1088/1367-2630/14/8/085001.
https://doi.org/10.1088/1367-2630/14/8/085001
[32] S. Diamond, S. Boyd, CVXPY: A Python-Embedded Modeling Language for Convex Optimization, JMLR 17 (2016) 5.
[33] A. Agrawal, R. Verschueren, S. Diamond, S. Boyd, A rewriting system for convex optimization problems, JJ. Control Decis. 5 (1) (2018) 42–60. doi:10.1080/23307706.2017.1397554.
https://doi.org/10.1080/23307706.2017.1397554
[34] J. E. Bourassa, R. N. Alexander, M. Vasmer, A. Patil, I. Tzitrin, T. Matsuura, D. Su, B. Q. Baragiola, S. Guha, G. Dauphinais, K. K. Sabapathy, N. C. Menicucci, I. Dhand, Blueprint for a Scalable Photonic Fault-Tolerant Quantum Computer, Quantum 5 (2021) 392. doi:10.22331/q-2021-02-04-392.
https://doi.org/10.22331/q-2021-02-04-392
[35] C. S. Hamilton, R. Kruse, L. Sansoni, S. Barkhofen, C. Silberhorn, I. Jex, Gaussian Boson Sampling, Phys. Rev. Lett. 119 (17) (2017) 170501. doi:10.1103/PhysRevLett.119.170501.
https://doi.org/10.1103/PhysRevLett.119.170501
[36] N. Quesada, J. M. Arrazola, N. Killoran, Gaussian boson sampling using threshold detectors, Phys. Rev. A 98 (6) (2018) 062322. doi:10.1103/PhysRevA.98.062322.
https://doi.org/10.1103/PhysRevA.98.062322
[37] H.-S. Zhong, H. Wang, Y.-H. Deng, M.-C. Chen, L.-C. Peng, Y.-H. Luo, J. Qin, D. Wu, X. Ding, Y. Hu, P. Hu, X.-Y. Yang, W.-J. Zhang, H. Li, Y. Li, X. Jiang, L. Gan, G. Yang, L. You, Z. Wang, L. Li, N.-L. Liu, C.-Y. Lu, J.-W. Pan, Quantum computational advantage using photons, Science 370 (6523) (2020) 1460. doi:10.1126/science.abe8770.
https://doi.org/10.1126/science.abe8770
[38] L. A. Howard, G. G. Gillett, M. E. Pearce, R. A. Abrahao, T. J. Weinhold, P. Kok, A. G. White, Optimal Imaging of Remote Bodies Using Quantum Detectors, Phys. Rev. Lett. 123 (14) (2019) 143604. doi:10.1103/PhysRevLett.123.143604.
https://doi.org/10.1103/PhysRevLett.123.143604
[39] K. Niwa, T. Numata, K. Hattori, D. Fukuda, Few-photon color imaging using energy-dispersive superconducting transition-edge sensor spectrometry, Sci. Rep. 7 (1) (2017) 45660. doi:10.1038/srep45660.
https://doi.org/10.1038/srep45660
[40] A. E. Lita, D. V. Reddy, V. B. Verma, R. P. Mirin, S. W. Nam, Development of Superconducting Single-Photon and Photon-Number Resolving Detectors for Quantum Applications, J. Lightwave Technol., JLT 40 (23) (2022) 7578–7597. https://opg.optica.org/jlt/abstract.cfm?uri=jlt-40-23-7578.
https://opg.optica.org/jlt/abstract.cfm?uri=jlt-40-23-7578
Cited by
[1] Lars S. Madsen, Fabian Laudenbach, Mohsen Falamarzi. Askarani, Fabien Rortais, Trevor Vincent, Jacob F. F. Bulmer, Filippo M. Miatto, Leonhard Neuhaus, Lukas G. Helt, Matthew J. Collins, Adriana E. Lita, Thomas Gerrits, Sae Woo Nam, Varun D. Vaidya, Matteo Menotti, Ish Dhand, Zachary Vernon, Nicolás Quesada, and Jonathan Lavoie, “Quantum computational advantage with a programmable photonic processor”, Nature 606 7912, 75 (2022).
[2] Miller Eaton, Amr Hossameldin, Richard J. Birrittella, Paul M. Alsing, Christopher C. Gerry, Hai Dong, Chris Cuevas, and Olivier Pfister, “Resolution of 100 photons and quantum generation of unbiased random numbers”, Nature Photonics 17 1, 106 (2023).
[3] Lorenzo Stasi, Gaëtan Gras, Riad Berrazouane, Matthieu Perrenoud, Hugo Zbinden, and Félix Bussières, “Fast High-Efficiency Photon-Number-Resolving Parallel Superconducting Nanowire Single-Photon Detector”, Physical Review Applied 19 6, 064041 (2023).
[4] Jaehak Lee, Nuri Kang, Seok-Hyung Lee, Hyunseok Jeong, Liang Jiang, and Seung-Woo Lee, “Fault-tolerant quantum computation by hybrid qubits with bosonic cat-code and single photons”, arXiv:2401.00450, (2023).
[5] Daiqin Su, Ish Dhand, and Timothy C. Ralph, “Universal quantum computation with optical four-component cat qubits”, Physical Review A 106 4, 042614 (2022).
[6] Joshua Combes and Austin P. Lund, “Homodyne measurement with a Schrödinger cat state as a local oscillator”, Physical Review A 106 6, 063706 (2022).
[7] Miller Eaton, Carlos González-Arciniegas, Rafael N. Alexander, Nicolas C. Menicucci, and Olivier Pfister, “Measurement-based generation and preservation of cat and grid states within a continuous-variable cluster state”, Quantum 6, 769 (2022).
[8] Prajit Dhara, Spencer J. Johnson, Christos N. Gagatsos, Paul G. Kwiat, and Saikat Guha, “Heralded Multiplexed High-Efficiency Cascaded Source of Dual-Rail Entangled Photon Pairs Using Spontaneous Parametric Down-Conversion”, Physical Review Applied 17 3, 034071 (2022).
[9] Italo Pereira Bezerra, Hilma M. Vasconcelos, and Scott Glancy, “Quadrature squeezing and temperature estimation from the Fock distribution”, Quantum Information Processing 21 11, 365 (2022).
[10] Josef Hloušek, Jan Grygar, Michal Dudka, and Miroslav Ježek, “High-resolution coincidence counting system for large-scale photonics applications”, Physical Review Applied 21 2, 024023 (2024).
[11] Christopher C. Gerry, Richard J. Birrittella, Paul M. Alsing, Amr Hossameldin, Miller Eaton, and Olivier Pfister, “Proposal for a quantum random number generator using coherent light and a non-classical observable”, Journal of the Optical Society of America B Optical Physics 39 4, 1068 (2022).
[12] Jakub Jirsa, Sergei Kulkov, Raphael A. Abrahao, Jesse Crawford, Aaron Mueninghoff, Ermanno Bernasconi, Claudio Bruschini, Samuel Burri, Stephen Vintskevich, Michal Marcisovsky, Edoardo Charbon, and Andrei Nomerotski, “Fast data-driven spectrometer with direct measurement of time and frequency for multiple single photons”, arXiv:2304.11999, (2023).
[13] Lorenzo Stasi, Patrik Caspar, Tiff Brydges, Hugo Zbinden, Félix Bussières, and Rob Thew, “High-efficiency photon-number-resolving detector for improving heralded single-photon sources”, Quantum Science and Technology 8 4, 045006 (2023).
[14] Aaron Z. Goldberg and Khabat Heshami, “Teleamplification on the Borealis boson-sampling device”, Physical Review A 108 6, 062606 (2023).
[15] Shuo Li, Wenchao Li, Vladislav V. Yakovlev, Allison Kealy, and Andrew D. Greentree, “En route to nanoscopic quantum optical imaging: counting emitters with photon-number-resolving detectors”, Optics Express 30 8, 12495 (2022).
[16] Tao Wang, Can Jiang, Junlong Zou, Hanxu Zhou, Xiao Lin, Hongsheng Chen, Gian Piero Puccioni, Gaofeng Wang, and Gian Luca Lippi, “Second-Order Correlation Function Supported Optical Sensing for Particle Detection”, IEEE Sensors Journal 21 18, 19948 (2021).
[17] M. Dryazgov, Yu. Korneeva, and A. Korneev, “Micrometer-Wide Nb N Strips for Photon-Number-Resolving Detection”, Physical Review Applied 19 3, 034067 (2023).
[18] Wenchao Li, Shuo Li, Timothy C. Brown, Qiang Sun, Xuezhi Wang, Vladislav V. Yakovlev, Allison Kealy, Bill Moran, and Andrew D. Greentree, “Estimation of the number of single-photon emitters for multiple fluorophores with the same spectral signature”, AVS Quantum Science 5 4, 041401 (2023).
[19] Rajveer Nehra, Miller Eaton, Olivier Pfister, and Alireza Marandi, “All-optical Quantum State Engineering for Rotation-symmetric Bosonic States”, arXiv:2105.11035, (2021).
[20] Ye-Chao Liu, Jiangwei Shang, and Xiangdong Zhang, “Efficient verification of entangled continuous-variable quantum states with local measurements”, Physical Review Research 3 4, L042004 (2021).
[21] Ling-Dong Kong, Tian-Zhu Zhang, Xiao-Yu Liu, Hao Li, Zhen Wang, Xiao-Ming Xie, and Li-Xing You, “Large-inductance superconducting microstrip photon detector enabling 10 photon-number resolution”, Advanced Photonics 6, 016004 (2024).
The above citations are from SAO/NASA ADS (last updated successfully 2024-05-24 22:08:40). The list may be incomplete as not all publishers provide suitable and complete citation data.
On Crossref’s cited-by service no data on citing works was found (last attempt 2024-05-24 22:08:39).
This Paper is published in Quantum under the Creative Commons Attribution 4.0 International (CC BY 4.0) license. Copyright remains with the original copyright holders such as the authors or their institutions.
- SEO Powered Content & PR Distribution. Get Amplified Today.
- PlatoData.Network Vertical Generative Ai. Empower Yourself. Access Here.
- PlatoAiStream. Web3 Intelligence. Knowledge Amplified. Access Here.
- PlatoESG. Carbon, CleanTech, Energy, Environment, Solar, Waste Management. Access Here.
- PlatoHealth. Biotech and Clinical Trials Intelligence. Access Here.
- Source: https://quantum-journal.org/papers/q-2024-05-23-1355/
- :is
- :not
- :where
- ][p
- $UP
- 08
- 1
- 10
- 100
- 11
- 12
- 13
- 133
- 14
- 15%
- 16
- 17
- 19
- 1995
- 1998
- 1999
- 20
- 2005
- 2006
- 2008
- 2009
- 2011
- 2012
- 2013
- 2014
- 2015
- 2016
- 2017
- 2018
- 2019
- 2020
- 2021
- 2022
- 2023
- 2024
- 22
- 23
- 24
- 25
- 26%
- 27
- 28
- 29
- 30
- 31
- 32
- 33
- 35%
- 36
- 37
- 39
- 40
- 51
- 521
- 66
- 7
- 75
- 8
- 9
- 95%
- 98
- a
- Aaron
- above
- ABSTRACT
- access
- Achieve
- achieved
- achieving
- acquisition
- Additionally
- ADvantage
- affiliations
- Alexander
- algorithm
- All
- allow
- Allowing
- allows
- am
- america
- among
- an
- analog
- analyse
- and
- Andrew
- Application
- applications
- applied
- approximate
- ARE
- AREA
- Array
- AS
- assigned
- astronomy
- At
- attempt
- austin
- author
- authors
- based
- Bay
- BE
- being
- Bill
- blueprint
- bodies
- boson
- Break
- brink
- brown
- by
- CAN
- Capacity
- carlos
- caspar
- CAT
- chen
- Chris
- Christopher
- Cluster
- codes
- COHERENT
- coincidence
- color
- Colorado
- comment
- Commons
- complete
- computation
- computational
- computer
- computing
- concise
- considerable
- continuous
- control
- conventional
- Conversion
- Convex
- copyright
- Correlation
- Counter
- counters
- counting
- custom
- data
- data-driven
- de
- demonstration
- Detection
- determined
- determining
- developed
- Development
- device
- Diamond
- direct
- discuss
- distribution
- does
- dong
- e
- Edge
- efficiency
- efficient
- enabling
- energy
- engineered
- Engineering
- Event
- express
- extensive
- extremely
- FAST
- faster
- feedback
- few
- finding
- First
- followed
- For
- found
- fox
- fpga
- Frequency
- from
- function
- gate
- generation
- generator
- Grid
- Hamilton
- Hardware
- harvard
- Have
- here
- High
- high-resolution
- Highlight
- holders
- However
- HTTPS
- hugo
- Hybrid
- i
- ideas
- IEEE
- image
- Imaging
- improving
- in
- information
- instantaneously
- Institute
- institutions
- interesting
- International
- ITS
- jacob
- Jan
- JavaScript
- jj
- Johnson
- jonathan
- joshua
- journal
- Kelley
- Kim
- known
- kok
- Kong
- kruse
- lab
- language
- large-scale
- Last
- Leave
- Lee
- levine
- Li
- License
- light
- lin
- List
- local
- Lolli
- Low
- mathematics
- matthew
- max-width
- May..
- measurement
- measurements
- measuring
- metal
- Metcalf
- methods
- Miller
- modeling
- Month
- multiphoton
- multiple
- Nam
- Nature
- New
- Nicolas
- no
- normal
- number
- numbers
- Nuri
- of
- Olivier
- on
- open
- operation
- opt
- Optical physics
- optimal
- optimization
- optimized
- or
- order
- original
- pages
- pairs
- Paper
- Parallel
- paris
- Paul
- Perform
- phd
- Photons
- Physics
- plato
- Plato Data Intelligence
- PlatoData
- potential
- Practical
- practice
- precise
- precisely
- preparation
- preservation
- problems
- process
- processing
- Processor
- programmable
- Progress
- property
- proposal
- provide
- providing
- published
- publisher
- publishers
- pulse
- Quantum
- quantum computational advantage
- Quantum computational advantage using photons
- Quantum Computer
- quantum computing
- Quantum Generation
- quantum information
- quantum systems
- quantum technology
- Qubit
- qubits
- R
- Rafael
- random
- range
- real
- real-time
- realistic
- recovery
- reducing
- references
- registered
- remains
- remote
- required
- Requirements
- requires
- research
- Resolution
- resolve
- resolving
- resource
- response
- review
- rewriting
- Richard
- rob
- Route
- s
- same
- scalable
- SCI
- Science
- Science and Technology
- scott
- sensitive
- sensor
- sensors
- show
- Signal
- signature
- single
- small
- smith
- Society
- Source
- Sources
- Spectral
- speed
- standards
- State
- States
- Stephen
- Still
- structure
- Successfully
- such
- suitable
- Sun
- superconducting
- Superconductivity
- Supported
- system
- Systems
- Technologies
- Technology
- that
- The
- their
- theory
- thesis
- they
- this
- thomas
- threshold
- Through
- till
- time
- Title
- to
- towards
- Transactions
- transformational
- transition
- Trevor
- unbiased
- under
- Universal
- university
- unparalleled
- updated
- URL
- us
- use
- used
- uses
- using
- Valley
- variable
- varun
- Verification
- vincent
- volume
- W
- wang
- want
- was
- we
- WELL
- which
- while
- white
- with
- within
- Woo
- Work
- works
- wu
- X
- x-ray
- xiao
- year
- You
- zephyrnet
- Zhao
- Zhong