Particle therapy – cancer treatment using beams of protons or heavier ions – provides highly conformal dose delivery and greater sparing of normal tissues than conventional photon-based radiotherapy. But for long-term cancer survivors, the risk of radiation-induced secondary cancer (SC) is important, and should be considered when selecting their treatment modality.
With epidemiological data scarce for newer treatments such as proton and carbon-ion therapy, a team headed up at the GSI Helmholtz Centre for Heavy Ion Research is developing a model to compare the SC risks between particle therapy modalities. The model, described by Antonia Hufnagl and colleagues in Medical Physics, could ultimately be incorporated into treatment planning systems to include SC risk as an additional optimization criterion.
Lethal versus carcinogenic events
SC risk models typically work by considering the balance between cell kill (leading to cancer suppression) and cell transformation (induction of mutations that eventually lead to cancer). The probability that an irradiated volume will develop cancer is defined using the linear–quadratic (LQ) model, which provides a simple relationship between cell survival and delivered photon dose.
In this study, the researchers used the local effect model (LEM) to predict the relative biological effectiveness (RBE) of SC induction after particle therapy. To account for the increased RBE of particle radiation, they replaced the photon LQ parameters in the risk model with the ion-beam LQ parameters predicted by the LEM. A key feature of their approach is the use of the LEM in both the cell killing and cancer induction terms.
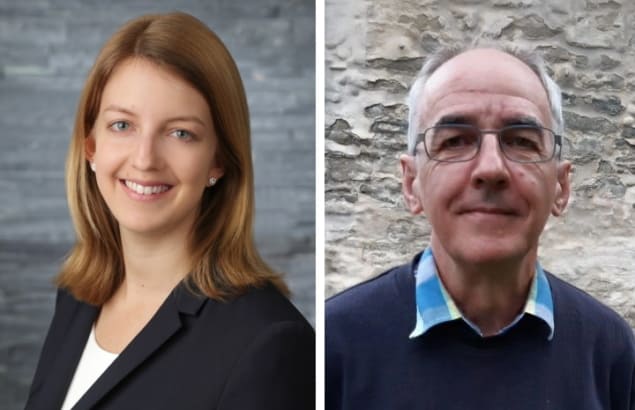
“The double use of the LEM reflects the competition between the two major processes determining SC development, namely cell transformation and cell kill,” explains senior author Michael Scholz. “With increasing dose and/or effectiveness, cell kill can supress the viability of transformed cells. This leads to a complex interplay, which cannot be simply reflected otherwise in a one-step procedure.”
To investigate which factors impact SC risk, the researchers used the TPS TRiP98 planning system to generate biologically optimized carbon-ion and proton treatment plans based on an idealized geometry. The plans irradiated a 4x4x4 cm target with a single particle beam or two opposing beams, with a 4x4x1 cm organ-at-risk (OAR) in front of the target. Due to uncertainties in the photon LQ parameters used as input for the LEM, they estimated proton-to-carbon ion risk ratios, rather than individual risk values.
For these idealized set-ups, the model did not show a clear preference for either protons or carbon ions, but revealed a complex dependence on various parameters. The reduced lateral scattering of carbon ions leads to a lower SC risk than protons in the entrance channel. However, carbon ions deposit a higher dose behind the target due to the fragmentation tail, increasing the SC risk for OARs behind the tumour after carbon-ion irradiation.
For single-beam plans, the total SC risk was roughly 1.5 times higher for carbon ions than for protons. With two opposing beams, the total SC risk was 1.16 times higher for protons, although this varied strongly depending on the spatial location of the assumed sensitive volume with respect to the target volume.
Tissue radiosensitivity (to photons) had a major impact on the SC risk ratio, with radioresistant OARs benefiting from carbon-ion treatment and sensitive OARs from proton beams. In contrast, the fractionation scheme had little impact on expected risk values.
Patient geometry
To investigate clinical scenarios, Scholz and colleagues estimated the SC risks for 10 prostate cancer patients previously treated with photon radiotherapy at Karolinska University Hospital. They generated treatment plans for the patients using two laterally opposed scanned proton and carbon-ion fields.
As seen previously, the fragmentation tail of carbon ions resulted in a large low-dose area behind the target. However, the high-dose target region was more conformal for the carbon-ion than the proton plans.
The team calculated the proton-to-carbon ion SC risk ratios for four OARs (bladder, rectum, bones and skin) for the 10 patients. For bone and skin, proton plans yielded a slightly higher SC risk than carbon-ion plans, with median risk ratios of 1.19 and 1.06 for bone and skin, respectively. For bladder and rectum, however, proton plans resulted in significantly lower SC risks, with risk ratios of 0.68 and 0.49 for bladder and rectum, respectively.
The researchers conclude that the insights gained by this model could help optimize future treatments. Currently, relative risk modelling is mainly suitable as a tool for comparing different treatment scenarios for different patient cohorts. But Scholz notes that incorporating such models into treatment planning for individual patients would be straightforward.
Carbon ion grid therapy spares healthy tissue
“It just requires running the planning for a given dose distribution with two different biological parameter sets representing the cell kill and the cell transformation process, respectively,” he explains. “Then, only some postprocessing of the resulting 3D effect distributions with standard mathematical tools is needed to derive the corresponding risk ratio distributions.”
The next step, he says, is to validate the model via comparison to clinical data. “Since at present these data are scarce, extension of the approach to also include photon treatments and determining the corresponding risk ratios of protons versus photons and carbon ions versus photons would be an important next step,” Scholz tells Physics World.