1Pritzker School of Molecular Engineering, University of Chicago, Chicago, IL, USA
2National Center for Computational Sciences, Oak Ridge National Laboratory, TN 37831, USA
3Department of Physics and Astronomy, Northwestern University, Evanston, IL 60208, USA
Find this paper interesting or want to discuss? Scite or leave a comment on SciRate.
Abstract
Driven quantum systems subject to non-Markovian noise are typically difficult to model even if the noise is classical. We present a systematic method based on generalized cumulant expansions for deriving a time-local master equation for such systems. This master equation has an intuitive form that directly parallels a standard Lindblad equation, but contains several surprising features: the combination of driving and non-Markovianity results in effective time-dependent dephasing rates that can be negative, and the noise can generate Hamiltonian renormalizations even though it is classical. We analyze in detail the highly relevant case of a Rabi-driven qubit subject to various kinds of non-Markovian noise including $1/f$ fluctuations, finding an excellent agreement between our master equation and numerically-exact simulations over relevant timescales. The approach outlined here is more accurate than commonly employed phenomenological master equations which ignore the interplay between driving and noise.
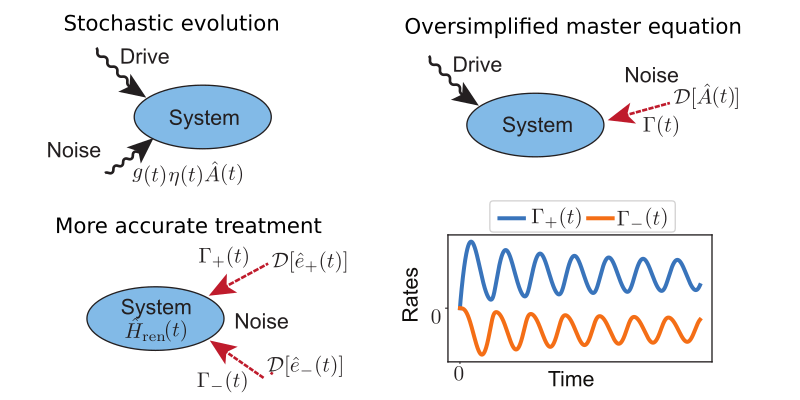
Popular summary
► BibTeX data
► References
[1] Vittorio Gorini, Andrzej Kossakowski, and E. C. G. Sudarshan. “Completely positive dynamical semigroups of n‐level systems”. Journal of Mathematical Physics 17, 821–825 (1976).
https://doi.org/10.1063/1.522979
[2] G. Lindblad. “On the generators of quantum dynamical semigroups”. Communications in Mathematical Physics 48, 119–130 (1976).
https://doi.org/10.1007/BF01608499
[3] J. R. Johansson, P. D. Nation, and Franco Nori. “Qutip: An open-source python framework for the dynamics of open quantum systems”. Computer Physics Communications 183, 1760–1772 (2012).
https://doi.org/10.1016/j.cpc.2012.02.021
[4] Heinz-Peter Breuer and Francesco Petruccione. “The theory of open quantum systems”. Oxford University Press, USA. (2002).
https://doi.org/10.1093/acprof:oso/9780199213900.002.14005
[5] Nicolas Didier, Eyob A. Sete, Joshua Combes, and Marcus P. da Silva. “ac flux sweet spots in parametrically modulated superconducting qubits”. Phys. Rev. Applied 12, 054015 (2019).
https://doi.org/10.1103/PhysRevApplied.12.054015
[6] Agustin Di Paolo, Thomas E. Baker, Alexandre Foley, David Sénéchal, and Alexandre Blais. “Efficient modeling of superconducting quantum circuits with tensor networks”. npj Quantum Information 7, 1–11 (2021).
https://doi.org/10.1038/s41534-020-00352-4
[7] M. A. Yurtalan, J. Shi, G. J. K. Flatt, and A. Lupascu. “Characterization of multilevel dynamics and decoherence in a high-anharmonicity capacitively shunted flux circuit”. Physical Review Applied 16, 054051 (2021).
https://doi.org/10.1103/PhysRevApplied.16.054051
[8] F. Setiawan, Peter Groszkowski, Hugo Ribeiro, and Aashish A. Clerk. “Analytic design of accelerated adiabatic gates in realistic qubits: General theory and applications to superconducting circuits”. PRX Quantum 2, 030306 (2021).
https://doi.org/10.1103/PRXQuantum.2.030306
[9] G. Ithier, E. Collin, P. Joyez, P. J. Meeson, D. Vion, D. Esteve, F. Chiarello, A. Shnirman, Y. Makhlin, J. Schriefl, and G. Schon. “Decoherence in a superconducting quantum bit circuit”. Phys. Rev. B 72, 134519 (2005).
https://doi.org/10.1103/PhysRevB.72.134519
[10] Alexandre Blais, Arne L. Grimsmo, Steven M. Girvin, and Andreas Wallraff. “Circuit quantum electrodynamics”. Reviews of Modern Physics 93, 025005 (2021).
https://doi.org/10.1103/RevModPhys.93.025005
[11] E. Paladino, Y. M. Galperin, G. Falci, and B. L. Altshuler. “1/f noise: Implications for solid-state quantum information”. Reviews of Modern Physics 86, 361 (2014).
https://doi.org/10.1103/RevModPhys.86.361
[12] E. Paladino, L. Faoro, G. Falci, and Rosario Fazio. “Decoherence and 1/f noise in josephson qubits”. Physical review letters 88, 228304 (2002).
https://doi.org/10.1103/PhysRevLett.88.228304
[13] Tobias Becker, Ling-Na Wu, and André Eckardt. “Lindbladian approximation beyond ultraweak coupling”. Physical Review E 104, 014110 (2021).
https://doi.org/10.1103/PhysRevE.104.014110
[14] Ryogo Kubo. “Generalized cumulant expansion method”. Journal of the Physical Society of Japan 17, 1100–1120 (1962).
https://doi.org/10.1143/JPSJ.17.1100
[15] N. G. Van Kampen. “A cumulant expansion for stochastic linear differential equations. i”. Physica 74, 215–238 (1974).
https://doi.org/10.1016/0031-8914(74)90121-9
[16] N. G. Van Kampen. “A cumulant expansion for stochastic linear differential equations. ii”. Physica 74, 239–247 (1974).
https://doi.org/10.1016/0031-8914(74)90122-0
[17] Ronald Forrest Fox. “Critique of the generalized cumulant expansion method”. J. Math. Phys. 17, 7 (1976).
https://doi.org/10.1063/1.523041
[18] Gulácsi, Balázs and Burkard, Guido. “Smoking-gun signatures of non-Markovianity of a superconducting qubit”. arXiv:2302.09092 (2023).
https://doi.org/10.48550/arXiv.2302.09092
arXiv:2302.09092
[19] J. Budimir and J. L. Skinner. “On the relationship between $T_1$ and $T_2$ for stochastic relaxation models”. Journal of Statistical Physics 49, 1029–1042 (1987).
https://doi.org/10.1007/BF01017558
[20] M. Aihara, H. M. Sevian, and J. L. Skinner. “Non-markovian relaxation of a spin-1/2 particle in a fluctuating transverse field: Cumulant expansion and stochastic simulation results”. Physical Review A 41, 6596–6601 (1990).
https://doi.org/10.1103/PhysRevA.41.6596
[21] Yuan-Chi Yang, S. N. Coppersmith, and Mark Friesen. “Achieving high-fidelity single-qubit gates in a strongly driven charge qubit with $1/f$ charge noise”. npj Quantum Information 5, 12 (2019).
https://doi.org/10.1038/s41534-019-0127-1
[22] Michael J. W. Hall, James D. Cresser, Li Li, and Erika Andersson. “Canonical form of master equations and characterization of non-markovianity”. Phys. Rev. A 89, 042120 (2014).
https://doi.org/10.1103/PhysRevA.89.042120
[23] John H. Reina, Luis Quiroga, and Neil F. Johnson. “Decoherence of quantum registers”. Phys. Rev. A 65, 032326 (2002).
https://doi.org/10.1103/PhysRevA.65.032326
[24] G. Massimo Palma, Kalle-Antti Suominen, and Artur Ekert. “Quantum computers and dissipation”. Proceedings of the Royal Society of London. Series A: Mathematical, Physical and Engineering Sciences 452, 567–584 (1996).
https://doi.org/10.1098/rspa.1996.0029
[25] Heinz-Peter Breuer, Bernd Kappler, and Francesco Petruccione. “Stochastic wave-function method for non-markovian quantum master equations”. Physical Review A 59, 1633 (1999).
https://doi.org/10.1103/PhysRevA.59.1633
[26] S. Chaturvedi and F. Shibata. “Time-convolutionless projection operator formalism for elimination of fast variables. Applications to Brownian motion”. Zeitschrift für Physik B Condensed Matter 35, 297–308 (1979).
https://doi.org/10.1007/BF01319852
[27] V. V. Dobrovitski, A. E. Feiguin, R. Hanson, and D. D. Awschalom. “Decay of rabi oscillations by dipolar-coupled dynamical spin environments”. Phys. Rev. Lett. 102, 237601 (2009).
https://doi.org/10.1103/PhysRevLett.102.237601
[28] Heinz-Peter Breuer, Elsi-Mari Laine, Jyrki Piilo, and Bassano Vacchini. “Colloquium: Non-markovian dynamics in open quantum systems”. Rev. Mod. Phys. 88, 021002 (2016).
https://doi.org/10.1103/RevModPhys.88.021002
[29] K. Rabenstein, V. A. Sverdlov, and D. V. Averin. “Qubit decoherence by gaussian low-frequency noise”. Journal of Experimental and Theoretical Physics Letters 79, 646–649 (2004).
https://doi.org/10.1134/1.1790024
[30] P. Facchi, D. A. Lidar, and S. Pascazio. “Unification of dynamical decoupling and the quantum zeno effect”. Phys. Rev. A 69, 032314 (2004).
https://doi.org/10.1103/PhysRevA.69.032314
[31] J-M Cai, Boris Naydenov, Rainer Pfeiffer, Liam P. McGuinness, Kay D. Jahnke, Fedor Jelezko, Martin B. Plenio, and Alex Retzker. “Robust dynamical decoupling with concatenated continuous driving”. New Journal of Physics 14, 113023 (2012).
https://doi.org/10.1088/1367-2630/14/11/113023
[32] Kevin C. Miao, Joseph P. Blanton, Christopher P. Anderson, Alexandre Bourassa, Alexander L Crook, Gary Wolfowicz, Hiroshi Abe, Takeshi Ohshima, and David D. Awschalom. “Universal coherence protection in a solid-state spin qubit”. Science 369, 1493–1497 (2020).
https://doi.org/10.1126/science.abc5186
[33] Haimeng Zhang, Bibek Pokharel, E. M. Levenson-Falk, and Daniel Lidar. “Predicting non-markovian superconducting-qubit dynamics from tomographic reconstruction”. Physical Review Applied 17, 054018 (2022).
https://doi.org/10.1103/PhysRevApplied.17.054018
[34] Richard Hartmann and Walter T. Strunz. “Accuracy assessment of perturbative master equations: Embracing nonpositivity”. Physical Review A 101, 012103 (2020).
https://doi.org/10.1103/PhysRevA.101.012103
[35] Dorit Aharonov, Alexei Kitaev, and Noam Nisan. “Quantum circuits with mixed states”. In Proceedings of the thirtieth annual ACM symposium on Theory of computing. Pages 20–30. (1998).
https://doi.org/10.48550/arXiv.quant-ph/9806029
arXiv:quant-ph/9806029
[36] John Watrous. “The theory of quantum information”. Cambridge University Press. USA (2018). 1st edition.
https://doi.org/10.1017/9781316848142
[37] Ziwen Huang, Pranav S Mundada, András Gyenis, David I Schuster, Andrew A Houck, and Jens Koch. “Engineering dynamical sweet spots to protect qubits from 1/f noise”. Physical Review Applied 15, 034065 (2021).
https://doi.org/10.1103/PhysRevApplied.15.034065
[38] Ziwen Huang, Xinyuan You, Ugur Alyanak, Alexander Romanenko, Anna Grassellino, and Shaojiang Zhu. “High-order qubit dephasing at sweet spots by non-gaussian fluctuators: Symmetry breaking and floquet protection”. Physical Review Applied 18, L061001 (2022).
https://doi.org/10.1103/PhysRevApplied.18.L061001
[39] M. A. Nielsen and I. L. Chuang. “Quantum computation and quantum information”. Cambridge University Press. Cambridge, UK (2000).
https://doi.org/10.1017/CBO9780511976667
Cited by
[1] Evangelos Vlachos, Haimeng Zhang, Vivek Maurya, Jeffrey Marshall, Tameem Albash, and E. M. Levenson-Falk, “Master equation emulation and coherence preservation with classical control of a superconducting qubit”, Physical Review A 106 6, 062620 (2022).
[2] Alessio Paviglianiti, Soumik Bandyopadhyay, Philipp Uhrich, and Philipp Hauke, “Absence of operator growth for average equal-time observables in charge-conserved sectors of the Sachdev-Ye-Kitaev model”, Journal of High Energy Physics 2023 3, 126 (2023).
[3] Ziwen Huang, Yunwei Lu, Anna Grassellino, Alexander Romanenko, Jens Koch, and Shaojiang Zhu, “Completely Positive Map for Noisy Driven Quantum Systems Derived by Keldysh Expansion”, arXiv:2303.11491, (2023).
The above citations are from SAO/NASA ADS (last updated successfully 2023-04-07 01:36:26). The list may be incomplete as not all publishers provide suitable and complete citation data.
On Crossref’s cited-by service no data on citing works was found (last attempt 2023-04-07 01:36:25).
This Paper is published in Quantum under the Creative Commons Attribution 4.0 International (CC BY 4.0) license. Copyright remains with the original copyright holders such as the authors or their institutions.
- SEO Powered Content & PR Distribution. Get Amplified Today.
- Platoblockchain. Web3 Metaverse Intelligence. Knowledge Amplified. Access Here.
- Source: https://quantum-journal.org/papers/q-2023-04-06-972/
- :is
- ][p
- 1
- 10
- 11
- 1996
- 1998
- 1999
- 2012
- 2014
- 2016
- 2018
- 2019
- 2020
- 2021
- 2022
- 2023
- 28
- 39
- 7
- 8
- 9
- a
- above
- ABSTRACT
- accelerated
- access
- accurate
- ACM
- affiliations
- Agreement
- alex
- Alexander
- All
- Although
- analysis
- analyze
- and
- Andrew
- annual
- applications
- applied
- approach
- ARE
- AS
- assessment
- astronomy
- At
- author
- authors
- average
- based
- BE
- between
- Beyond
- Bit
- boris
- Break
- Breaking
- by
- cambridge
- CAN
- case
- Center
- charge
- chicago
- Christopher
- combination
- comment
- commonly
- Commons
- Communications
- complete
- completely
- computation
- computer
- computers
- computing
- Condensed matter
- Consisting
- contains
- continuous
- control
- copyright
- Daniel
- data
- David
- Derived
- Design
- detail
- detailed
- difficult
- directly
- discuss
- driven
- driving
- dynamics
- e
- edition
- effect
- Effective
- embracing
- energy
- Engineering
- environments
- equations
- Erika
- Even
- example
- excellent
- expansion
- FAST
- Features
- field
- Find
- finding
- fluctuations
- FLUX
- For
- form
- found
- Framework
- from
- Gary
- Gates
- General
- generate
- generators
- Growth
- Hall
- harvard
- here
- High
- highly
- holders
- HTTPS
- hugo
- i
- implications
- in
- Including
- information
- institutions
- interesting
- International
- intuitive
- IT
- Japan
- JavaScript
- John
- Johnson
- journal
- Koch
- laboratory
- Last
- Leave
- Li
- License
- List
- London
- map
- Marcus
- mark
- Martin
- master
- math
- mathematical
- Matter
- max-width
- method
- Michael
- mixed
- model
- models
- Modern
- molecular
- Month
- more
- motion
- nation
- National
- negative
- networks
- New
- Nicolas
- Noise
- oak
- Oak Ridge National Laboratory
- of
- on
- open
- open source
- operator
- original
- outlined
- Oxford
- oxford university
- Paolo
- Paper
- Parallels
- Peter
- physical
- Physics
- plato
- Plato Data Intelligence
- PlatoData
- positive
- present
- press
- Proceedings
- Projection
- protect
- protection
- provide
- published
- publisher
- publishers
- Python
- Quantum
- quantum information
- quantum systems
- Qubit
- qubits
- Rates
- realistic
- references
- registers
- relationship
- relevant
- remains
- Results
- review
- Reviews
- Richard
- royal
- s
- School
- Science
- SCIENCES
- Sectors
- Series
- Series A
- several
- show
- Signatures
- Simple
- simulation
- Society
- Spin
- standard
- States
- statistical
- strongly
- subject
- Successfully
- such
- suitable
- superconducting
- surprising
- sweet
- Symposium
- Systems
- that
- The
- their
- theoretical
- Title
- to
- types
- typically
- Uk
- under
- university
- University of Chicago
- updated
- URL
- USA
- various
- volume
- W
- WELL
- which
- with
- Work
- works
- wu
- year
- You
- zephyrnet