
Physicists at Harvard University in the US have created a novel strongly interacting quantum liquid known as a Laughlin state in a gas of ultracold atoms for the first time. The state, which is an example of a fractional quantum Hall (FQH) state, had previously been seen in condensed-matter systems and in photons, but observations in atoms had been elusive due to stringent experimental requirements. Because atomic systems are simpler than their condensed-matter counterparts, the result could lead to fresh insights into fundamental physics.
“Some of the most intriguing phenomena in condensed-matter physics emerge when you confine electrons in two dimensions and apply a strong magnetic field,” explains Julian Léonard, a postdoctoral researcher in the Rubidium Lab at Harvard and the lead author of a paper in Nature on the new work. “For example, the particles can behave collectively as if they have a charge that is only a fraction of the elementary charge – something that does not occur anywhere else in nature and is even ruled out by the Standard Model for all fundamental particles.”
The way in which such fractional charges arise is still not fully understood because it is difficult to study solid-state systems at an atomic scale. This is why it is so desirable to study the behaviour of FQHs in synthetic quantum systems such as cold atoms, which act as quantum simulators for more complex condensed-matter phenomena.
In the latest study, for example, members of the Harvard team directly observed particles in their atomic system moving around each other in a circular pattern, rather like “dancers in a waltz”, says Léonard. “This vortex motion is too small to see in a solid-state sample, but we are able to resolve it in our experiment,” he tells Physics World.
Making atoms behave more like electrons
To create the Laughlin state, Léonard and colleagues used overlapping laser beams to form a periodic lattice potential made from light. They then placed atoms into each lattice site and tuned the parameters of the beams so that the atoms were free to “hop” between sites. This setup mimics the periodic potential experienced by electrons in a crystalline solid, Léonard explains. “The only difference is that our artificial crystal is more than 1000 times larger, so we can observe and control each ‘electron’ with an optical microscope,” he says.
One major challenge for the Harvard team was to mimic the electrons’ response to magnetic fields. While negatively charged electrons experience a force (the Lorenz force) in a direction perpendicular to their motion when placed in a magnetic field, the atoms that play the role of electrons in the new platform are electrically neutral, meaning that this force is absent. The researchers therefore had to “trick” the atoms into behaving more like electrons in a magnetic field.
To do this, they relied on the fact that when electrons circumnavigate a magnetic field, their wavefunction acquires a phase. This is known as the Aharonov–Bohm effect, and Léonard explains that they were able to create an equivalent in cold atoms. “In our experiments, we made use of several laser beams that applied exactly this phase to the atoms’ wavefunctions,” he says.
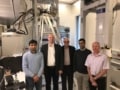
Holes reveal first fractional quantization
Possibility of observing anyons
The team also faced challenges in creating the strong, precisely engineered magnetic field required to observe FQH states, which had previously remained out of reach for laboratory experiments, Léonard adds. “We have now shown for the first time that it is possible to study strongly correlated systems under a magnetic field in a quantum simulator,” he says. “It is therefore now possible to study such states on a microscopic level and glean new insights into them. We may even discover completely new phenomena that have so far remained inaccessible.”
While the number of atoms in the FQH Laughlin state observed by the researchers is small, at just two atoms across 16 lattice sites, the team believes the system size could be increased. “A larger system will allow us to gain an even better view of the physics that underlies the FQH effect and one aspect we are particularly excited to observe are the excitations in such systems,” says Léonard. “These are believed to be neither fermions nor bosons, but so-called anyons, which are a completely new type of particle that fall outside our usual classification of quantum statistics.”
- SEO Powered Content & PR Distribution. Get Amplified Today.
- PlatoData.Network Vertical Generative Ai. Empower Yourself. Access Here.
- PlatoAiStream. Web3 Intelligence. Knowledge Amplified. Access Here.
- PlatoESG. Automotive / EVs, Carbon, CleanTech, Energy, Environment, Solar, Waste Management. Access Here.
- BlockOffsets. Modernizing Environmental Offset Ownership. Access Here.
- Source: https://physicsworld.com/a/fractional-quantum-hall-state-appears-in-ultracold-atoms/
- :is
- :not
- :where
- 16
- a
- Able
- absent
- ABSTRACT
- Acquires
- across
- Act
- Adds
- All
- allow
- also
- an
- and
- anywhere
- appears
- applied
- Apply
- ARE
- arise
- around
- artificial
- AS
- aspect
- At
- atom
- author
- BE
- because
- been
- believed
- believes
- Better
- between
- but
- by
- CAN
- challenge
- challenges
- charge
- charged
- charges
- classification
- cold
- colleagues
- collectively
- completely
- complex
- control
- could
- create
- created
- Creating
- Crystal
- difference
- difficult
- dimensions
- direction
- directly
- discover
- do
- does
- due
- each
- effect
- electrons
- else
- emerge
- Equivalent
- Even
- exactly
- example
- excited
- experience
- experienced
- experiment
- experiments
- Explains
- faced
- fact
- Fall
- far
- field
- Fields
- First
- first time
- For
- Force
- form
- fraction
- fractional
- Free
- fresh
- from
- fully
- fundamental
- Gain
- GAS
- goldman
- had
- Hall
- harvard
- harvard university
- Have
- he
- HTML
- HTTPS
- if
- image
- in
- inaccessible
- increased
- information
- insights
- interacting
- into
- intriguing
- issue
- IT
- ITS
- jpg
- just
- known
- lab
- laboratory
- larger
- laser
- lasers
- latest
- lead
- Level
- light
- like
- Liquid
- made
- Magnetic field
- major
- manipulated
- max-width
- May..
- meaning
- Members
- Microscope
- model
- more
- most
- motion
- moving
- Nature
- negatively
- Neither
- Neutral
- New
- New Platform
- novel
- now
- number
- observe
- of
- on
- ONE
- only
- Other
- our
- out
- outside
- Paper
- parameters
- particularly
- Pattern
- peculiar
- periodic
- phase
- Photons
- Physics
- Physics World
- platform
- plato
- Plato Data Intelligence
- PlatoData
- Play
- possible
- potential
- precisely
- previously
- Quantum
- quantum systems
- rather
- reach
- realized
- remained
- required
- Requirements
- researcher
- researchers
- response
- result
- reveal
- Role
- ruled
- says
- Scale
- see
- seen
- setup
- several
- shown
- simpler
- simulator
- site
- Sites
- Size
- small
- So
- so Far
- solid
- something
- standard
- State
- States
- statistics
- Still
- strong
- strongly
- Study
- such
- synthetic
- system
- Systems
- team
- tells
- than
- that
- The
- The State
- their
- Them
- then
- therefore
- they
- this
- thumbnail
- time
- times
- to
- too
- true
- two
- type
- under
- understood
- university
- us
- use
- used
- View
- was
- Way..
- we
- were
- when
- which
- while
- why
- will
- with
- Work
- world
- You
- zephyrnet