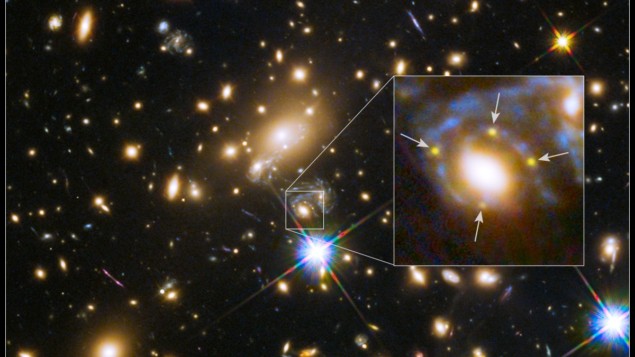
A study of how light from a distant supernova was gravitationally lensed as it travelled to Earth has been used to calculate a new value for the Hubble constant – an important parameter that describes the expansion of the universe. While this latest result has not surprised astronomers, similar observations in the future could help us understand why different techniques have so far yielded very different values for the Hubble constant.
The universe has been expanding since it was created in the Big Bang 13.7 billion years ago. In the 1920s, the American astronomer Edwin Hubble observed that galaxies further away from Earth appear to be moving away from Earth faster than galaxies that are closer to us. He did this by measuring the redshift of the light from these galaxies — which is the stretching of the wavelength of light that occurs when an object recedes from an observer.
The linear relationship between distance and speed that he measured is described by the Hubble constant and astronomers have since developed several techniques to measure it.
Astronomers are puzzled, however, because different measurements have delivered very different values for the Hubble constant. Measurements of the cosmic microwave background (CRB) radiation by the European Space Agency’s Planck satellite give a value of about 67 km/s/Mpc. However, measurements involving observations of the type 1a supernovae done by the SH0ES collaboration give a value of about 73 km/s/Mpc. The uncertainties in these measurements are about 1–2%, so there is a clear tension between the two techniques. Astronomers want to know why, and to find out they are developing new ways to measure the Hubble constant.
Now, astronomers have measured the Hubble constant using light from a supernova that exploded 9.34 billion years ago. On its way to Earth, the light passed through a galaxy cluster and was deflected by the cluster’s immense gravitational field, which focused the light towards Earth. This effect is called gravitational lensing.
Lumpy mass distribution
The lumpy distribution of mass in the cluster created a complex gravitational field that sent the supernova’s light along several different paths towards Earth. When the supernova was first observed in 2014, it appeared as four points of light. As the four points faded, a fifth appeared 376 days later. This light was delayed by the longer path it had taken through the cluster.
During those 376 days the universe had expanded, which means that the wavelength of the late arriving light was redshifted. By measuring this extra redshift, a team led by Patrick Kelly of the University of Minnesota was able to calculate the Hubble constant. Using several different mass distributions models for the clusters, the team came up with values for the constant of either 64.8 km/s/Mpc or 66.6 km/s/Mpc.
The supernova time-delay measurement would at first glance seems to favour Planck’s value of the Hubble constant over SH0ES. However, previous time delay measurements of quasar light observed by the H0LiCOW collaboration give a value 73.3 km/s/Mpc – so closer to SH0ES.
While this might seem confusing, Kelly’s colleague Tommaso Treu of the University of California, Los Angeles points out that the latest results are not surprising.
“They are not very different,” he says. “Within the uncertainties, this new measurement is consistent with all three [Planck, SH0ES and H0LiCOW].”
Sherry Suyu of the Max Planck Institute for Astrophysics in Germany, who leads the H0LiCOW project and was not involved in these new time-delay measurements, also doesn’t necessarily see a paradox.
Future promise
“This value [from the supernova] is from a single lens system, and given its error bars, the measurement is statistically consistent with the results from H0LiCOW’s lensed quasars,” she says.
The uncertainty in the supernova time-delay measurement comes is related to how mass is distributed in the galaxy – how much dark matter and baryonic (normal) matter is present and how it is spread throughout the cluster. Kelly and Treu’s team used a variety of models, and the differences between the models forms a large part of the uncertainty in their values for the Hubble constant.
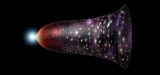
Finding a consistent constant
“The precision of the low Hubble constant measurements presented here just isn’t enough to argue against the higher SH0ES value,” says Daniel Mortlock of Imperial College, London, who was also not involved in the research.
Still, Mortlock thinks that this calculation of the Hubble constant from the time-delay measurement of a supernova is a landmark. So far, only a couple of lensed supernovae have been discovered, but in the coming years when the Vera C. Rubin Observatory in Chile, which sports a giant 8.4-metre survey telescope, comes online the number of lensed supernova discoveries should dramatically increase.
“Lovely” work
“Overall I think it’s a lovely piece of work to make this measurement, but perhaps the most exciting aspect of this is future promise, since surveys like Rubin will discover many more systems of this type,” Mortlock says.
With increased numbers of lensed supernovae will come greater precision in the measurements of the Hubble constant, which will help reduce the error bars and confirm whether these data support the Planck or SH0ES results. Some theorists have even suggested that new physics may be required to explain the Hubble tension, assuming that it is real and not an unrecognized systematic error in the observations.
“Clearly more precision is needed to contribute to the resolution of the Hubble tension,” concludes Treu. “But this is an important first step.”
The research is described in Science.
- SEO Powered Content & PR Distribution. Get Amplified Today.
- PlatoAiStream. Web3 Data Intelligence. Knowledge Amplified. Access Here.
- Minting the Future w Adryenn Ashley. Access Here.
- Buy and Sell Shares in PRE-IPO Companies with PREIPO®. Access Here.
- Source: https://physicsworld.com/a/gravitational-lensing-of-supernova-yields-new-value-for-hubble-constant/
- :has
- :is
- :not
- $UP
- 13
- 2014
- 66
- 67
- 7
- 8
- 9
- a
- Able
- About
- AC
- against
- ago
- All
- along
- also
- American
- an
- and
- Angeles
- appear
- appeared
- ARE
- argue
- arriving
- AS
- aspect
- At
- away
- background
- bars
- BE
- because
- been
- between
- Big
- Big Bang
- Billion
- but
- by
- calculate
- california
- called
- came
- Chile
- clear
- closer
- Cluster
- collaboration
- colleague
- College
- come
- comes
- coming
- complex
- Confirm
- confusing
- consistent
- constant
- contribute
- could
- Couple
- created
- Dark
- Dark matter
- data
- Days
- delay
- Delayed
- delivered
- described
- developed
- developing
- DID
- differences
- different
- discover
- discovered
- distance
- distributed
- distribution
- distributions
- Doesn’t
- done
- dramatically
- earth
- Edwin
- effect
- either
- enough
- error
- European
- Even
- exciting
- expanded
- expanding
- expansion
- Explain
- extra
- far
- faster
- field
- Find
- First
- focused
- For
- forms
- four
- from
- further
- future
- Galaxies
- Galaxy
- Germany
- giant
- GitHub
- Give
- given
- Glance
- gravitational
- greater
- had
- Have
- he
- help
- here
- higher
- How
- However
- HTML
- HTTPS
- Hubble
- Hubble Constant
- i
- image
- images
- immense
- Imperial
- Imperial College
- important
- in
- Increase
- increased
- information
- Institute
- involved
- involving
- issue
- IT
- ITS
- jpg
- just
- Know
- landmark
- large
- Late
- later
- latest
- Leads
- Led
- light
- like
- London
- longer
- los
- Los Angeles
- Low
- make
- many
- Mass
- Matter
- max
- max-width
- May..
- means
- measure
- measurement
- measurements
- measuring
- might
- models
- more
- most
- moving
- much
- necessarily
- needed
- New
- normal
- number
- numbers
- object
- of
- on
- online
- only
- or
- out
- over
- Paradox
- parameter
- part
- passed
- path
- perhaps
- Physics
- Physics World
- piece
- plato
- Plato Data Intelligence
- PlatoData
- points
- Precision
- present
- presented
- previous
- project
- promise
- Quasar
- real
- reduce
- related
- relationship
- required
- research
- Resolution
- result
- Results
- satellite
- says
- Science
- see
- seem
- seems
- sent
- several
- she
- should
- shown
- similar
- since
- single
- So
- so Far
- some
- Space
- speed
- Sports
- spread
- Step
- Study
- supernova
- support
- surprised
- surprising
- Survey
- system
- Systems
- taken
- team
- techniques
- telescope
- than
- that
- The
- The Future
- their
- There.
- These
- they
- think
- Thinks
- this
- those
- three
- Through
- throughout
- thumbnail
- time
- to
- towards
- true
- two
- type
- ucla
- uncertainties
- Uncertainty
- understand
- Universe
- university
- University of California
- us
- used
- using
- value
- Values
- variety
- very
- want
- was
- Way..
- ways
- when
- whether
- which
- while
- WHO
- why
- will
- with
- Work
- world
- would
- years
- yielded
- yields
- zephyrnet